Human Induced Pluripotent Stem Cells
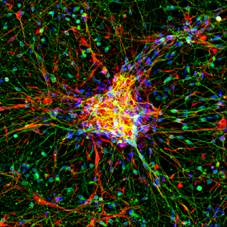
The latest development is the use of human induced pluripotent stem cells (iPSCs) as model for neurotoxicity testing. One particular advantage iof the use of human cells is the elimination of the need for interspecies translation. Additionally, multiple types of human iPSCs are available that can be used to assess specific types of toxicity (for example by using dopaminergic neurons) and/or grow into sophisticated networks of mixed neuronal nature. These human iPSC-derived, spontaneously active, neuronal networks effectively capture the complexity of the nervous system and represent the current state of the art for in vitro neurotoxicity testing. Currently, human iPSCs are used in several of our projects to investigate the effects of chemicals/drugs on (the development of) neuronal (network) functionality, changes in intracellular calcium levels and/or neurotransmitter release (Hondebrink et al., 2017; Kasteel and Westerink, 2017; Tukker et al., 2016; Tukker er al., 2018).
Primary Rat Cortical Cultures
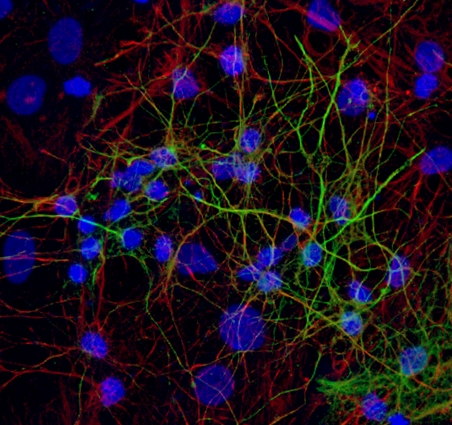
Primary cortical cultures are the current standard to capture the complexity of the nervous system in an in vitro model for neurotoxicity testing. These cortical cultures are heterogeneous and consist of different types of inhibitory GABA-ergic and excitatory glutamatergic neurons and supportive cells, e.g. astrocytes. In vitro, these cells grow into complex network of specialized, highly connected and structured cells that develop spontaneous network activity after 1 week in culture (Dingemans et al., 2016). As such, these cells are useful for investigating effects of chemicals on (the development of) neuronal (network) functionality, changes in intracellular calcium levels and/or neurotransmitter release (de Groot et al., 2016; Duarte et al., 2017; Hondebrink et al., 2016, 2017). Recently, cortical cultures grown on multi-electrode arrays have been used for food safety testing (Kasteel and Westerink, 2017; Nicolas et al., 2014).
Neurospheres
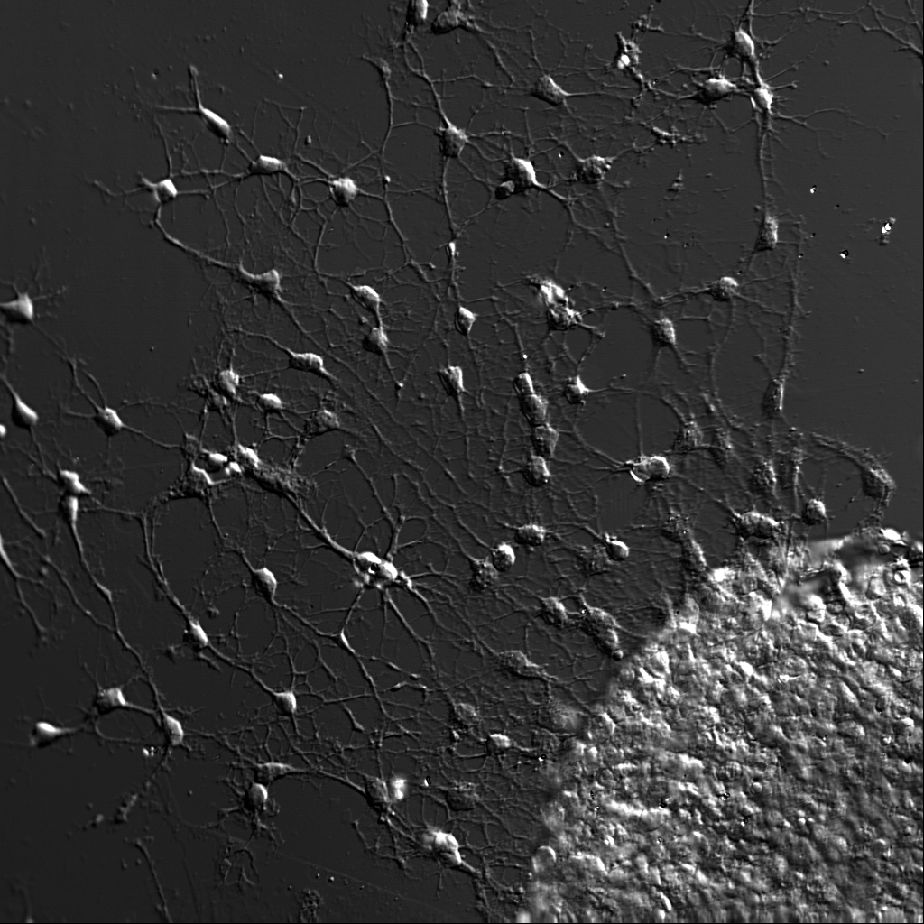
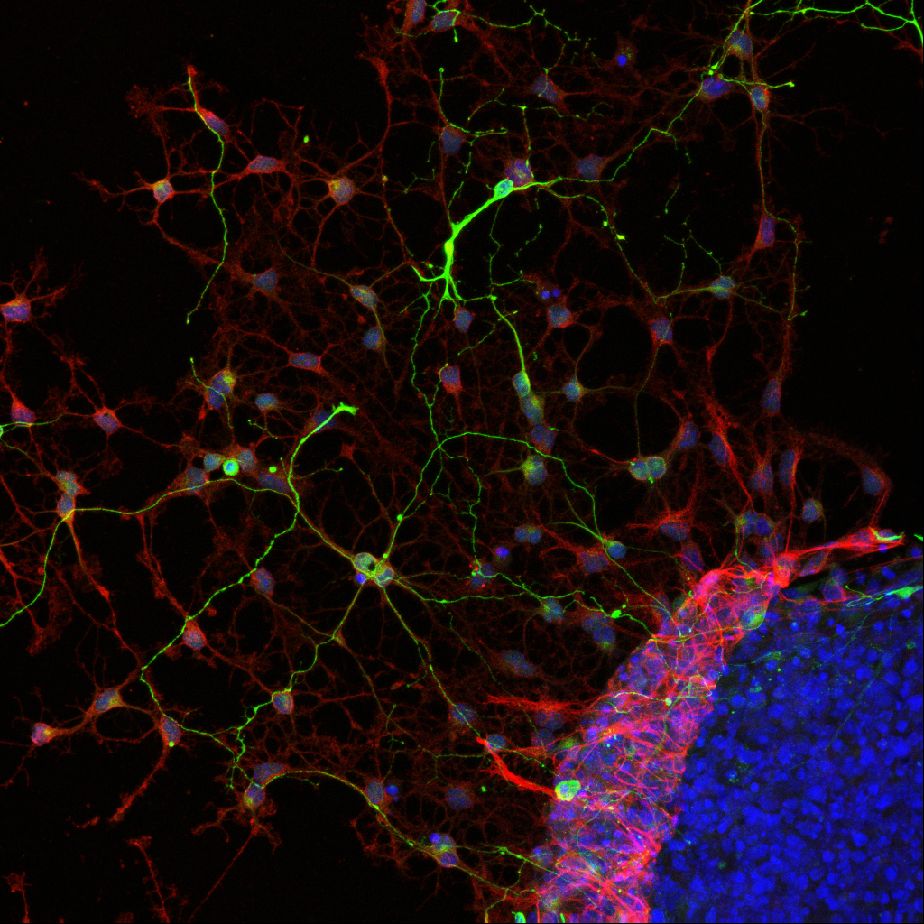
The period of rapid brain development is the most sensitive phase with respect to in vivo neurotoxicity. Developing neurospheres, derived from embryonic neural progenitor cells, allow for studying neuronal growth, migration and differentiation as well as apoptosis (all essential parameters for brain development) in vitro for up to several days or even weeks. Neurospheres are a heterogeneous cell model consisting of several neuronal and supportive cell types that exert cell-cell communication. Neurospheres are sensitive to oxidative stress and is has been shown that known developmental neurotoxicants, e.g., methylmercury, affect cell viability, the relative abundance of neurons and migration distance in this model. As neurospheres resemble in vivo neurodevelopment, are sensitive to known developmental neurotoxicants they are a suitable for studying developmental neurotoxicity (Moors et al., 2009, Environ Health Perspect.).
Differentiated neurospheres form a heterogeneous network of neuronal and supportive cells. These heterogeneous networks can be used to assess effects of chemical exposure on neuronal function and vulnerability. Our experiments revealed that differentiated networks display a wide variety of calcium signals, including transient calcium increases in response to a range of neurotransmitters (a.o. ATP, acetylcholine and glutamate) as well as spontaneous network activity (de Groot et al., 2014 Toxicol. Sci.) and sensitivity to chemical insults (Gassmann et al., 2014).
Hippocampal Slices
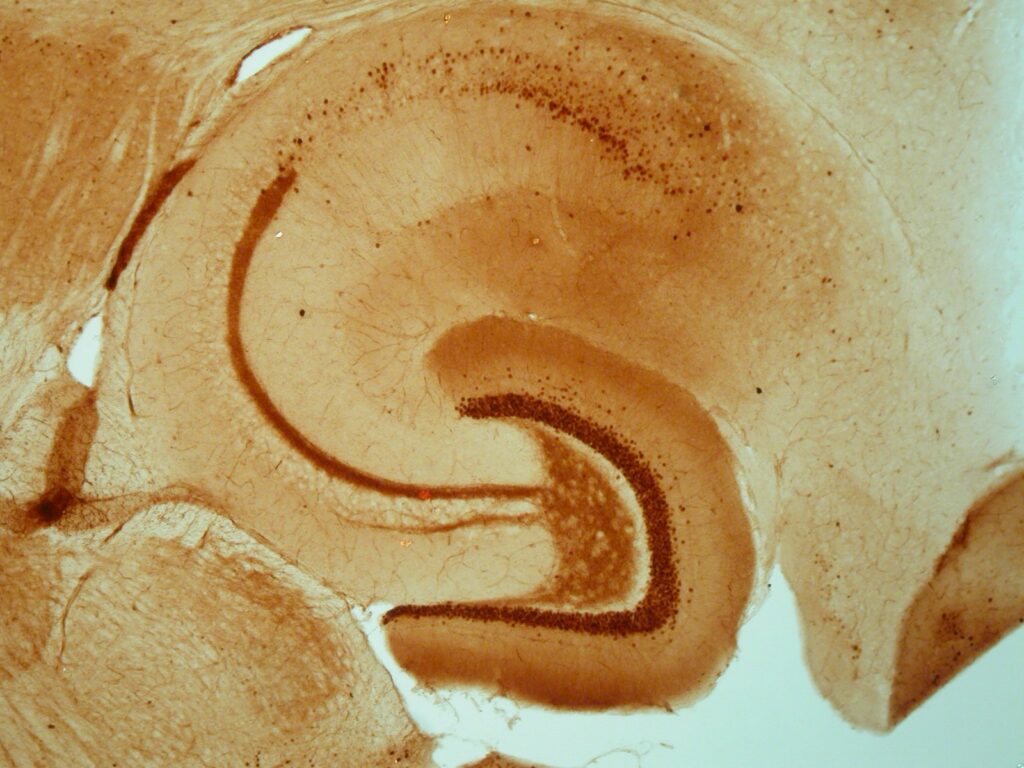
Though it is somewhat more complex to study cellular and molecular mechanisms in hippocampal slices, slices are suited to detect effects on synaptic plasticity. The sophisticated interactions that normally occur in the brain are virtually absent in most in vitro models, but largely retained in slices. Consequently, hippocampal slices can be used in specific cases as ex vivo preparation to validate observed in vitro findings with respect to the in vivo situation (Dingemans et al., 2007; Westerink et al., 2012; Hendriks et al., 2015).
Oocytes
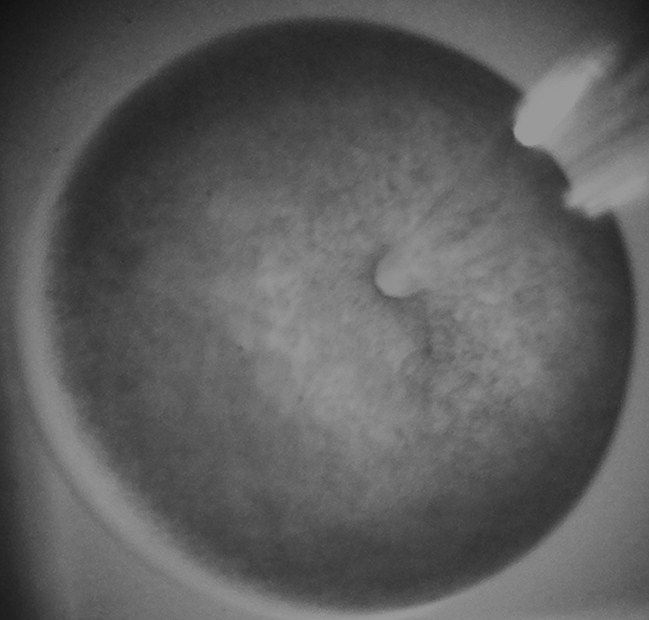
Xenopus laevis oocytes were introduced as a research model in the late 1950s. Oocytes efficiently translate cDNA that is injected into the nucleus into RNA and subsequently into functional proteins. The newly synthesized proteins are then transported to their expected location, e.g., the cell membrane (Brown, 2004, J. Biol. Chem.). Importantly, the oocytes are in this way capable of expressing distinct subtypes of neurotransmitter receptors. Moreover, the oocytes are easy to manipulate due to their relatively large size (~ 1 mm) and allow for electrophysiological recording of receptor-mediated ion currents. As a result, Xenopus laevis oocytes are nowadays widely used as model for the heterologues expression of neuronal proteins, like neurotransmitter receptors. These neurotransmitter receptor-expressing oocytes are a valuable tool to study the effects of toxicants on receptor function (see e.g., Antunes Fernandes et al., 2010; Hendriks et al., 2010, 2012; Hondebrink et al., 2011, 2013, 2015; van Kleef et al., 2008).
PC-12 Cells
PC12 cells (Greene and Tischler, 1976, PNAS) derived from a tumour in the adrenal medulla of the rat and thus are chromaffin-like cells. Importantly, PC12 cells and chromaffin cells have the same embryonic origin as sympathetic (dopaminergic) neurons. Like adrenal chromaffin cells, PC12 cells synthesize, store and release catecholamines in a calcium-dependent manner. PC12 cells express a variety of receptors, including nicotinic acetylcholine receptors and ionotropic ATP receptors. PC12 cells contain large dense-core vesicles (LDCVs) that store catecholamines and small synaptic-like vesicles (SSLVs) that store acetylcholine. In our hands, LDCVs of PC12 cells contain 99% dopamine and <1% noradrenaline (Westerink et al., 2002, Br. Res.).
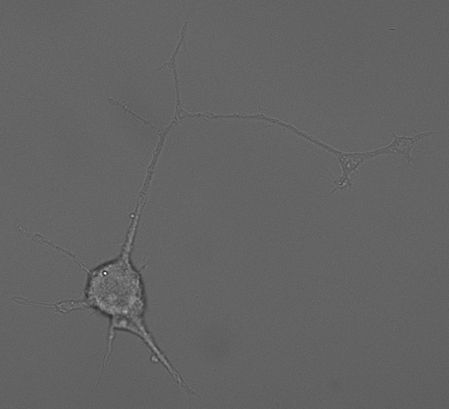
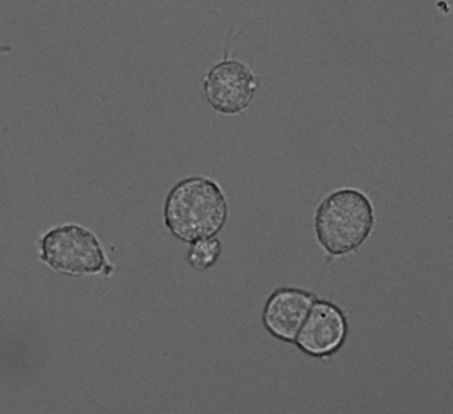
PC12 cells are easily cultured and can be differentiated into a more neuronal phenotype using e.g., NGF, or into a more endocrine cell-type using glucocorticoids like dexamethasone. In undifferentiated cells the number of releasable vesicles as well as the amount of catecholamines per vesicle is limited. As a result, the secretory response is subjected to strong rundown during successive stimulation (Westerink et al., 2000, BBRC). PC12 cells are therefore often differentiated with dexamethasone to increase the amount of catecholamines per vesicle and increases the number of releasable vesicles during successive stimulations (Westerink and Vijverberg, 2002, J.Neurochem.). Fortunately, these dexamethasone-differentiated PC12 cells are comparable with undifferentiated PC12 cells with respect to calcium channel expression patterns. Dexamethasone-differentiated PC12 cells have thus become a valuable and valid tool that allows for reliable measurements of exocytosis.
PC12 cells can also be pre-exposed to dexamethasone, l-DOPA (dopamine precursor) or iron to evoke chemically-induced oxidative stress. These stressed PC12 cell models show increased vulnerability to rotenone-induced cytotoxicity and may be valuable to investigate how increased cellular stress influences neurotoxic outcome (de Groot and Westerink, 2014).
Consequently, PC12 cells have become a popular model to study calcium homeostasis, neurotransmitter synthesis and neurotransmitter secretion (exocytosis) and are extensively used in neurotoxicological studies (Westerink and Ewing, 2008, Acta Physiol.).